DNA
microarray technology and bioinformatics have enabled some
advances in the understanding and manipulation of gene behavior
in simple, developmental models. These advances have medical
implications, especially with regard to the treatment of
carcinogenesis.
There remains, nevertheless, major problems, such as those that
pertain to the genomic control of mutation --- especially of
hypermutation --- which prevent a complete understanding and
hence a comprehensive, effective application of the new
biotechnologies and the imaginative creation of new ones. This
is especially the situation in the context of investigating,
unstable gene behavior within complex, developmental systems,
both in vivo and in vitro.
One
can re-conceptualize biological investigations / experiments in
order to solve these problems.
Through my extensive and diversified training and experience in
the genetic / epigenetic control of mutation and in vitro
development, as well as in its theoretical aspects, I can define
those problems and provide in depth solutions, which have
significant implications for developmental genetics.
In general, such solutions would require new, related paradigms,
suggesting investigations along new avenues. This would result
in comprehensive, in depth, and thus effective, medical and
agricultural applications.
As
a Consultant to
certain companies or laboratories, I could comprehensively
illustrate the problems and in turn develop solutions---perhaps
in collaboration with scientists who would be open to exploring
new paradigms---that would ultimately give forth powerful
applications.
With regard to in vitro developmental systems, I have a broad
background in various facets of genetics, including the
transgenic transformation of plants for disease resistance.
My
specialty has
been in developing procedures for overcoming recalcitrant
organogenesis and embryogenesis from plant tumor (callus) in
tissue culture of various plant species, including trees, an
important requirement, for recovering transgenic plantlets by
way of transformed callus in vitro. Pine, bean, and tobacco were
amongst the plants investigated in tissue culture by this author
(Lieber, 1980a, 1995,
1996).
The photographs (Figures 1a thru 6 and Figures 15a thru 19) are examples of my
experiments leading to successful plantlet, bud, and embyogenic
development at high frequency in vitro, where factors inhibiting
in vitro development were repeatedly overcome.
Such experiments are the results of a successful application of a principle within a
comprehensive theory pertaining to non-uniform forces (stress) presented to the organism in different ways
and their role in biological development (Lieber, 1996,
1998a, 1998b,
1998c, 2000,
2001a, 2001b) [
and weblinks].
This principle becomes apparent, among other ways, through the
manifestation of a universal, dimensionless biological constant,
first noted by the author (Lieber,
1998a)
This constant, numerically equivalent to the Golden Ratio,
1.618, was found to compose the universal dimensional constants of
physics. Among other matters, this constant, which is universal, defines or manifests
an underlying developmental, dynamical pattern in all physical
and biological phenomena.
For example, this dimensionless constant is readily seen as
contributing to the Planck Length. The latter is an universal dimensional
constant which refers to the connection between gravitational
force and quantum processes in a nearly infinitesimal region of
space-time and suggests, because of its dimensionless component,
a particular dynamic pattern, a spiral generation, operating
through that connection.
On the much higher organizational level or scale of biological
phenomena, this dynamical pattern is readily manifested in the
spiral/vortical morphology of many plants and animals, and their
parts, e.g., pinecones. Photographs (Figures 9a thru 14) show
this particular morphology.
Adaptively responsive hypermutation to stress, manifested as non-uniform forces, is a phenomenon
that is also predicted by the principle and its biological
constant. Using the fungus Aspergillus
nidulans, experimental conditions and genetic strains were
created by the author that demonstrated
this type of mutagenesis in response to a physical stress in the fungus and its importance for
development (Lieber, 1975c,
1976b, 1998b)
[and weblinks].
The importance of this research was described in a personal letter by the Nobel
Laureate, Dr. Barbara McClintock. Photographs show some examples of this phenomenon
(Figures 7 & 8 ). See
McClintock Letter
Later
research by the author demonstrated adaptively responsive hypermutation
to nutritional stress in bacteria. Such stress could be manifested or
presented as non-uniform force-magnitudes. See Lieber, 1980b;
Lieber & Persidock, 1983; Lieber, 1989,
1990, 1998b,1998c,
2000, 2001a
[and weblinks].
This research predicted problems and implied solutions to such (Lieber, 1989) which are currently being
addressed in plant genetics research (Lieber, 2005). Moreover,
the findings, using bacteria, were later confirmed by other
scientists. (References are given in my publications.)
Such research has begun to open up a whole new way of looking at
mutagenesis, especially its relationship to disease and to the
development of beneficial crops and trees.
However, to be even more effective, the medical and agricultural
sciences require newer, more completed, developed approaches
utilizing new and more completed theoretical models or
paradigms. Most likely, the universal dimensionless constant would be involved.
A comprehensive, new theory is needed, not just facets or parts
of such. Without new theory, on which scientific progress has
been based, the biological sciences will eventually stagnate;
consequently, they will be unable to address key problems,
calling for creative approaches, thus jeopardizing the future of
human progress and development.
Because
of my broad experience in the biological sciences, creativity,
and in-depth approach, I have provided and am able to provide
new, effective theoretical
perspectives and breakthroughs on research issues in biology, especially as they pertain to
mutation, gene function, development, and adaptation. In this
way, I could, as a Consultant, provide a valuable service in any
research endeavor.
For example, I can provide detailed protocols---as opposed to
conventional protocols---that enable a high frequency of plant
development (regeneration) from plant neoplasm in culture.
If you want to review such a protocol for a given plant species,
this would be provided for at a very reasonable fee.
I can also provide
on-site
advice/support at
your laboratory or agency.
For more infornation please refer to Biography
of Michael Lieber.
I can be contacted at Genadyne Consulting, Phone: (510) 526-4224
E-mail: michaellieber@juno.com Michael M. Lieber,
Ph.D. [TOP]
CAPTIONS
for PHOTOGRAPHS
Figure
1a - Very small
embryonic pine-plantlet (top center) emerging from brown inhibited
callus (neoplasm) derived from a pine needle.
Figure 1b - Pine bud emerging from callus derived from a pine
needle.
Figure 1c - Pine shoot growing from callus
derived from a pine needle.
Figure 1d - Small male pine-cone developed
from pine-needle callus.
Figure 1e - Pine shoot growing form callus derived from pine
needle.
Figure 2 - Bean
plantlets and buds emerging from callus having an immature-embryo
source.
Figure 3a - Bean embryos regenerated from
somatic callus derived from a shoot apex. Many embryos have
produced plantlet-shoots.
Figure 3b - Embryogenesis from bean callus
producing bean shoots. Callus was derived from the meristem of a
bud apex.
Figure 3c - Embryogenesis from another bean callus poducing
bean shoots
Figure 4 - Buds in various developmental
stages emerging from callus derived from a shoot apex of the green
bean.
Figure 5 - Bean plantlets regenerated from meristemic callus
of a shoot apex.
Figure 6 - Buds
and plantlets regenerated from meristemic bean-callus of a shoot
apex.
Figure 7 - An example of hypermutation in the
fungus, Aspergillus nidulans, cultured under stress.
Figure 8 - Adaptively responsive hypermutation
in Aspergillus cultured
under an increased-temperature stress.
Figure 9a -
Pine cone displaying seed nodules arranged in logarithmic
spirals.
Figure 9b - Bottom of a pine cone displaying
a spiral morphology.
Figures - 10a
and 10b - Vortical cacti.
Figure 11 - Cactus with a spiral morphology.
Figure 12 - Helical tendril of a vine.
Figure 13 - Two roses, each with a vortical
design.
Figure 14 - A gastropod shell with a spiral
morphology.
Figure 15a -
Deteriorated pine buds from needle callus. Pinus taeda.
Figure 15b -
Non-Deteriorated pine bud from needle callus. Pinus taeda.
Figure 16 -
Small, clustered pine buds from needle callus and tiny pinelet
from callus on far left side . Pinus muricata.
Figure 17 -
Small pine buds from needle callus and possible, tiny pinelets
from callus in central region of photo. Pinus muricata. A
definite pine plantlet with branches is located on the left side
of the brown callus.
Figure 18 -
Tiny pinelet from needle callus in
middle of culture, with two other possible pinelets nearby. Buds
from callus also present. Pinus muricata.
Figure 19 -
Bean plantlets
regenerated from callus cultured on medium with methylglyoxal but
without ascorbic acid.
MICHAEL
LIEBER's SCIENTIFIC ARTICLES and REPORTS New_Pespective_on_Plant_Tissue_Culture
Evolution-Environmentally-Responsive-karyotypic-mutator.htm
Dimensionless-Biological-Constant-φ
Spiral-Dimensionless-Constant-in-Physical-Constants
Bohm-and-Inner-Ordered-Mutation-A-Perspective-on-Discontinuity
Pineapple [TOP
|
Click
To Enlarge Photos
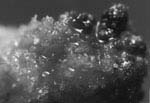
Figure 1a
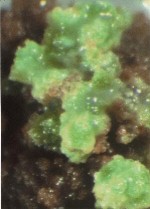
Figure 1b
Figure 1c
Figure 1d
Figure 1e
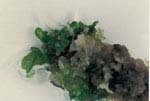
Figure 2
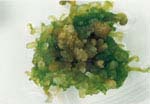
Figure 3a
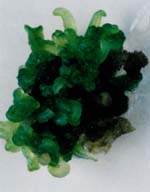
Figure 3b
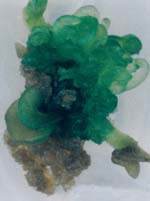
Figure 3c
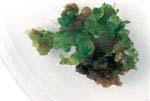
Figure 4
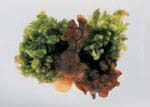
Figure 5
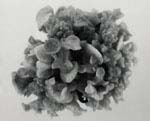
Figure 6
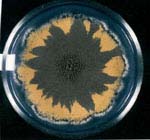
Figure 7
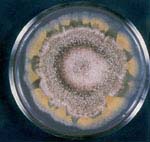
Figure 8
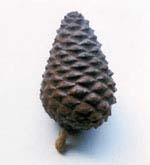
Figure 9a
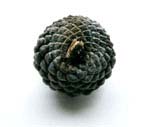
Figure 9b
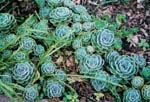
Figure 10a
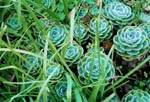
Figure 10b
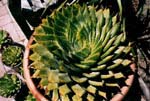
Figure 11
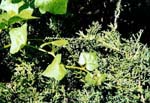
Figure 12
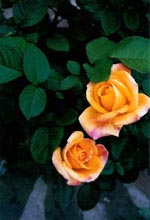
Figure 13
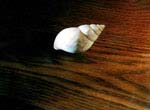
Figure 14
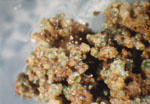
Figure 15a
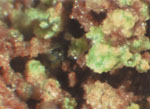
Figure 15b
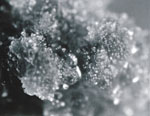
Figure 16
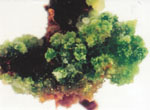
Figure 17
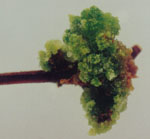
Figure 18
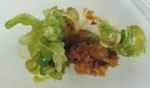
Figure 19 |